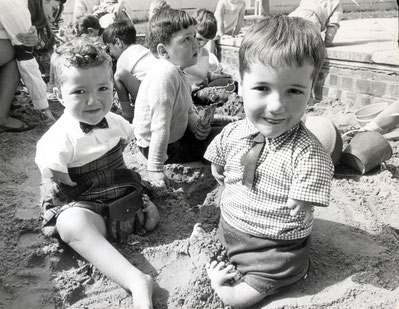
Thalidomide is a sedative drug developed by the German pharmaceutical company Chemie Grünenthal in the late 1950s to treat nausea. Despite a lack of rigorous scientific research, Grünenthal assured that thalidomide was safe for pregnant women to consume, and it was consequently prescribed to treat morning sickness and pregnancy-associated insomnia (The New York Times, 2013). In 1961, evidence came to light linking prenatal thalidomide toxicity to a range of congenital malformations, known as teratogenesis, in German babies (Bastow et al., 2017). It is estimated that 15,000 children worldwide were born with malformations attributable to thalidomide. Many newborns died a few days after birth while surviving babies most commonly suffered from Phocomelia (shortening of the arms and legs) (Thalidomide victims association of Canada, 2014). Following what was one of the most horrifying episodes in medical history, thalidomide was banned in 1962. However, new therapeutic effects of the drug have since been discovered, such as alleviating symptoms of leprosy and treating certain cancers (The New York Times, 2013). Since its scientific rehabilitation in the 1990s, thalidomide and its analogues have become the primary treatments for multiple myeloma. However, the reintroduction of thalidomide has been accompanied by several ethical issues. In Brazil, where the drug is used extensively to treat leprosy, hundreds of thalidomide children have been born since 2005 (MacDougall, 1998). In this essay, I will outline the molecular mechanisms linking prenatal thalidomide exposure to teratogenesis, and describe the pathways through which thalidomide can have such diverse physiological effects. I will also argue that, provided they remain stringently regulated, thalidomide and its analogues have the potential to improve the lives of millions of people worldwide, and thus they should not be banned.
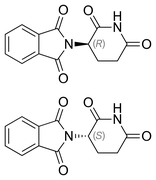
Thalidomide and its analogues lenalidomide and pomalidomide are small molecules synthetically derived from glutamic acid which are collectively known as immune-modulating drugs (IMiDs). Aside from their extensive use to treat leprosy and multiple myeloma, IMiDs have also been prescribed against a wide range of other diseases, such as Multiple Sclerosis, Tuberculosis, Crohn's Disease and AIDS (Stewart, 2014). Meanwhile, the range of birth defects attributed to thalidomide is as widespread as its medical benefits; thalidomide embryopathy can affect the limbs, face, eyes, ears, genitalia and internal organs, including the heart, kidney, and gastrointestinal tract, with an infant mortality of around 40%, likely attributed to internal organ damage (Vargesson, 2015). For many years, the biochemical nature of these diverse effects was largely unknown. However, recent studies have greatly enhanced our understanding of the molecular mechanisms of thalidomide and other IMiDs.
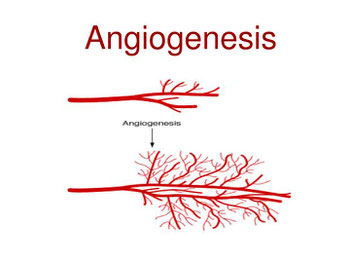
The anti-angiogenic properties of IMiDs were first discovered in 1992. Angiogenesis is the physiological process through which new blood vessels sprout from pre-existing vasculature, which is essential for tumour growth and metastasis (D’Amato et al., 1994). Using an aortic ring assay as an angiogenesis model, studies showed that thalidomide could starve blood flow to cells, giving it potential anti-cancer properties (Price et al., 2002). Thalidomide inhibits angiogenesis by trans-activating the enzyme acid sphingomyelinase (SMase), which in turn catalyses the breakdown of sphingomyelin into ceramide and phosphorylcholine. Ceramide inhibits the vascular endothelial growth factor receptor (VEGFR) and fibroblast growth factor receptor (FGFR) signalling pathways, both of which play central roles in angiogenesis (Yabu et al., 2005). Thalidomide also suppresses angiogenesis by interacting with cereblon (CRBN), thereby inhibiting the E3 ubiquitin-ligase activity of the CRBN/DDB-1/Cul4 complex. The direct ubiquitination targets of this complex have not been fully elucidated, however, studies indicate that the antagonism of cereblon by thalidomide reduces the levels of FGF8 and FGF10 (Kawamura et al., 2014). By limiting VEGF and FGF activities, thalidomide also induces loss of newly formed blood vessels during critical stages in limb development, causing cell death and teratogenicity.
Surprisingly, the interaction of lenalidomide with CRBN stimulates the CRBN/DDB-1/Cul4 E3 ubiquitin ligase to target the transcriptional regulator Ikaros for degradation. The degradation of Ikaros enhances IL-2 expression, which promotes differentiation of T cells and stimulates natural killer (NK) cell-mediated killing of multiple myeloma cells (Ito et al., 2010) (Lu et al., 2014). The inhibition of CRBN by thalidomide has been shown to be necessary but insufficient to induce teratogenicity in Zebrafish. In humans, CRBN mutations cause autosomal recessive mental retardation, but are not associated with limb defects, indicating that the combined effects of multiple pathways contribute to teratogenesis (Davies et al., 2001).
Indeed, thalidomide, lenalidomide and pomalidomide have been found to also contribute to teratogenesis by inducing oxidative stress. IMiDs are bioactivated by horseradish peroxidase to generate reactive oxygen species (ROS), which cause oxidative damage to DNA. The induction of oxidative stress in the developing limb buds suppresses NF-κB activity, culminating in the downregulation of FGF and the induction of apoptosis, contributing to teratogenesis (Parman et al., 1999). Lastly, IMiDs can intercalate DNA at GC-rich sequences, stimulating epigenetic silencing of GC-rich promoters by DNA methylation and histone acetylation. This inhibits the expression of FGF-2, affecting angiogenesis and embryonic limb development (Shortt et al., 2013). Therefore, the anti-angiogenic, pro-apoptotic, oxidative and epigenetic effects combine to induce teratogenicity.
Finally, thalidomide and its analogues are also potent inhibitors of the inflammatory response, making them effective treatments against inflammatory conditions such as leprosy and tuberculosis (TB). Leprosy and TB patients exhibit elevated cellular levels of TNF-α, a pro-inflammatory signalling protein, and IMiDs accelerate the degradation of TNF-α mRNA (Moreira et al., 1993).
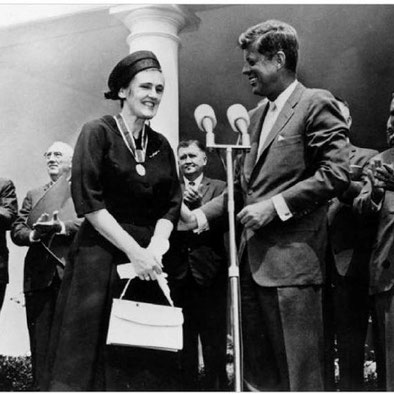
Dr Frances Kelsey being awarded the President's Award For Distinguished Federal Service from John F. Kenedy in 1962.
59 years after the scandal, the ethical issues surrounding thalidomide continue to shape medical ethics and drug administrative laws. In the late 1950s, thalidomide was deemed so safe that it was available to Germans without the need for a prescription. However, in 1960, Dr Frances Kelsey of the Food and Drug Administration (FDA) became concerned with the effect thalidomide might have on a developing foetus and ensured that the drug never entered the American market. Consequently, of the 15,000 cases of thalidomide babies, only 17 were in the USA (The New York Times, 2013). In addition to the congenital malformations, early patients began to notice unsuspected side effects to the nervous system attributed to thalidomide. A significant ethical issue is the fact that there was insufficient knowledge about thalidomide at the time of its launch. The drug hadn’t been thoroughly tested, with a complete absence of animal testing (Annas and Elias, 1999). Following the global banning of thalidomide, more rigorous drug approval regulations were passed by the FDA, including animal testing as a mandatory pre-clinical standard (Morusupali et al., 2016). When discussing the ethical issues surrounding thalidomide it is important two consider two ethical ideologies: Deontology and Utilitarianism. Deontology states that the correct course of action is solely dependent on one’s duties and obligations, while utilitarianism supports the course of action that brings the largest increase in utility (The Medic Portal, 2019). The intention behind marketing thalidomide for pregnant women was to alleviate morning sickness. Therefore, the act of prescribing the drug to pregnant women can be considered ethical in regards to the deontological stand point. Meanwhile, according to utilitarianism, marketing thalidomide at the time was unethical since it caused harm to thousands of babies.
Since its reinstatement in the 1990s, thalidomide has been one of the most restricted drugs ever to be distributed, with female patients having to submit to pregnancy tests before taking it (Thalidomide victims association of Canada, 2014). Although thalidomide babies continue to be born - particularly in Brazil, where the drug is used extensively to treat leprosy - the overall benefit achieved by its rehabilitation outweighs the harm it has caused. Stricter regulation of drug distributions and improved communication between patients and prescribing doctors could prevent further teratogenesis cases in the following years.
To conclude, thalidomide is a sedative drug that was developed in the late 1950s and is best known for causing a world-wide tragedy. Thousands of babies born in the early 1960s to mothers who consumed thalidomide during pregnancy had congenital malformations. It is now understood that these malformations occurred as a result of inhibition of blood vessel formation during embryonic development. This inhibitory function also makes thalidomide an effective anti-cancer drug. Additionally, thalidomide has anti-inflammatory properties and can be used to treat inflammatory diseases such as leprosy. The potential for thalidomide to cause damage to the developing foetus remains an important ethical concern in modern medicine and strict regulations are essential to prevent thalidomide-attributed teratogenesis in future years.
References:
- 2013. [online] Available at: <https://www.youtube.com/watch?v=41n3mDoVbvk&t=14s> [Accessed 18 March 2020].
- Annas, G. and Elias, S., 1999. Thalidomide and the Titanic: reconstructing the technology tragedies of the twentieth century. American Journal of Public Health, 89(1), pp.98-101.
- Bastow, B. and Isaacs, C., 2017. Teratology and Drug Use During Pregnancy. Medscape,.
- The Medic Portal. 2019. Complete Guide To Medical Ethics. [online] Available at: <https://www.themedicportal.com/blog/complete-guide-medical-ethics/> [Accessed 18 March 2020].
- Davies, F., Raje, N., Hideshima, T., Lentzsch, S., Young, G., Tai, Y., Lin, B., Podar, K., Gupta, D., Chauhan, D., Treon, S., Richardson, P., Schlossman, R., Morgan, G., Muller, G., Stirling, D. and Anderson, K., 2001. Thalidomide and immunomodulatory derivatives augment natural killer cell cytotoxicity in multiple myeloma. Blood, 98(1), pp.210-216.
- DʼAmato, R., Loughnan, M., Flynn, E. and Folkman, J., 1994. Thalidomide Is an Inhibitor of Angiogenesis. Retina, 16(3), p.268.
- Ito, T., Ando, H., Suzuki, T., Ogura, T., Hotta, K., Imamura, Y., Yamaguchi, Y. and Handa, H., 2010. Identification of a Primary Target of Thalidomide Teratogenicity. Science, 327(5971), pp.1345-1350.
- Kawamura, Y., Yamashita, T., Yamauchi, T., Matsumoto, K. and Sato, K., 2014. Effects of thalidomide on Fgf8, Bmp4 and Hoxa11 expression in the limb bud in Kbl:JW rabbit embryos. Congenital Anomalies, 54(1), pp.54-62.
- Lu, G., Middleton, R., Sun, H., Naniong, M., Ott, C., Mitsiades, C., Wong, K., Bradner, J. and Kaelin, W., 2013. The Myeloma Drug Lenalidomide Promotes the Cereblon-Dependent Destruction of Ikaros Proteins. Science, 343(6168), pp.305-309.
- MacDougall, D., 1998. The rehabilitation of thalidomide. J Int Assoc Physicians AIDS Care, (Jan;4(1):), pp.26-33.
- Moreira, A., Sampaio, E., Zmuidzinas, A., Frindt, P., Smith, K. and Kaplan, G., 1993. Thalidomide exerts its inhibitory action on tumor necrosis factor alpha by enhancing mRNA degradation. The Journal of Experimental Medicine, 177(6), pp.1675-1680.
- Morusupali, R., 2016. he tragedy of Thalidomide and the failure of animal testing. Environmental Conversation and Human health-Challenges and strategies,.
- Parman, T., Wiley, M. and Wells, P., 1999. Free radical-mediated oxidative DNA damage in the mechanism of thalidomide teratogenicity. Nature Medicine, 5(5), pp.582-585.
- Price, D., Ando, Y., Kruger, E., Weiss, M. and Figg, W., 2002. 5´-OH-Thalidomide, a Metabolite of Thalidomide, Inhibits Angiogenesis. Therapeutic Drug Monitoring, 24(1), pp.104-110.
- Shortt, J., Hsu, A. and Johnstone, R., 2013. Thalidomide-analogue biology: immunological, molecular and epigenetic targets in cancer therapy. Oncogene, 32(36), pp.4191-4202.
- Stephens, T., Bunde, C. and Fillmore, B., 2000. Mechanism of action in thalidomide teratogenesis. Biochemical Pharmacology, 59(12), pp.1489-1499.
- Stephens, T., Bunde, C. and Fillmore, B., 2000. Mechanism of action in thalidomide teratogenesis. Biochemical Pharmacology, 59(12), pp.1489-1499.
- Stewart, A., 2014. How Thalidomide Works Against Cancer. Science, 343(6168), pp.256-257.
- Vargesson, N., 2015. Thalidomide-induced teratogenesis: History and mechanisms. Birth Defects Research Part C: Embryo Today: Reviews, 105(2), pp.140-156.
- Thalidomide.ca. 2014. What Is Thalidomide? | Thalidomide. [online] Available at: <https://thalidomide.ca/en/what-is-thalidomide/> [Accessed 18 March 2020].
- Xue, H., Fu, W. and Cui, H., 2015. High-dose thalidomide increases the risk of peripheral neuropathy in the treatment of ankylosing spondylitis. Neural Regen Res., 10(5): 814–818., pp.814–818.
- Yabu, T., Tomimoto, H., Taguchi, Y., Yamaoka, S., Igarashi, Y. and Okazaki, T., 2005. Thalidomide-induced antiangiogenic action is mediated by ceramide through depletion of VEGF receptors, and is antagonized by sphingosine-1-phosphate. Blood, 106(1), pp.125-134.